Customizable soft biofunctionalized alginate microcarriers for stem cells cultivation in suspension bioreactors
Authors
Michael M. Gepp1,2, Petra Geßner3, Isabelle Sébastien1,2, Karina Ivaskevica1,2, Julia C. Neubauer1,2 and Heiko Zimmermann1,4,5
- Fraunhofer Institute for Biomedical Engineering IBMT, Sulzbach, Germany
- Fraunhofer Project Center for Stem Cell Process Engineering, Würzburg, Germany
- Alginatec GmbH, Riedenheim, Germany
- Molecular and Cellular Biotechnology/Nanotechnology, Saarland University, Saarbrücken, Germany
- Facultad de Ciencias del Mar, Universidad Católica del Norte, Coquimbo, Chile
Introduction
Three-dimensional (3D) cell culture technology is becoming increasingly important in research and the biotechnology industry. In particular, the expansion and differentiation of pluripotent stem cells on an industrial scale is the focus of many academic and industrial research groups. Although optimised protocols and culture media for lineage-specific 3D cultivation already exist, further developments in biomaterials and cell scaffolds are required. It is known that microcarriers can significantly increase the productivity of the bioprocess due to the higher surface to volume ratio (Figure 1a). For example, less cell culture medium is required, and more cells can be produced in a single bioreactor system. However, there are few commercially available soft microcarrier systems for stem cells. It is known that the mechanical properties of the biomaterial, such as elasticity, have a significant impact on cell culture behaviour. Therefore, alginate microcarriers with tuneable mechanical properties are advantageous for novel cell models in tissue engineering as they better mimic the physiological properties of the in vivo situation (Figure 1b). In this application note, we describe the properties of soft alginate microcarriers and their integration into human induced pluripotent stem cell (hiPSC) expansion processes using the CERO suspension bioreactor system.
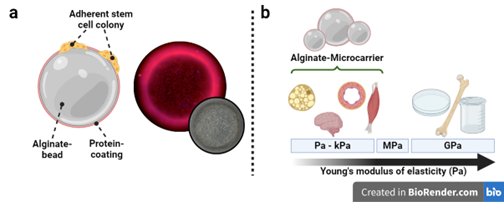
Figure 1: Alginate microcarriers. (a) Right: Schematic drawing of an idealized microcarrier with its elements: alginate beads made from UHV- alginate, the protein coating (e.g. Matrigel™ or Collagen I) for cell attachment. Left: Fluorescence image of alginate microcarrier confluently grown with hiPSC (stained cytoplasm and nuclei), inset: phase contrast image. (b) Alginate microcarriers can accommodate most cell types relevant to soft tissue engineering. Typical labware such as plastic dishes are out of range in terms of the Young’s modulus of tissues.
Materials and Methods
Alginate Microcarrier
Alginate microcarrier production (all steps performed at Alginatec GmbH, Riedenheim) involved the extraction and purification of ultra-high viscous (UHV) alginates from dried brown algae Lessonia nigrescens and Lessonia trabeculata, according to a quality-controlled process developed from [4]. Sterile alginates of both types were mixed in equal volumes, and spherical beads were produced using a coaxial air flow droplet generator. Alginates droplets were gelled in a crosslinking bath containing 20 mM BaCl2. The beads were then modified to immobilise crucial biochemical molecules, allowing for stem cell adhesion and colony formation (see also [1] and [2]). In the first step, the carboxyl groups of the alginate beads were activated using aqueous carbodiimide chemistry. In the second incubation step, Matrigel™ was covalently coupled to the alginate surface (see Figure 1). After incubation and thorough washing, we characterized alginate microcarriers coated with Matrigel™. We assessed their functionality, sterility, size, and growth area per volume. The microcarriers were then stored at 4°C in an isotonic NaCl solution until usage. We measured the mechanical properties of the alginate microcarriers using the nanoindentation system PAVONE (Optics11life).
a
b
c
Figure 2: 3D cultivation of the UKBi005-A cell line in a suspension bioreactor and on alginate microcarriers. (a) The total cell count and (b) the viability of the cell culture after four days of cultivation. The results showed no correlation between Young's modulus and cell behavior within the tight range of 2.49 to 4.44kPa. The seeding density was 6.0 x 104 cells/cm2 cell scaffold sample. (c) FACS analysis of the UKBi005-A cell line after 3D cultivation on 3D cell scaffolds prepared from differently composed alginate mixtures. The percentage of positive cells in which the two pluripotency markers SSEA4 and OCT-3/4 and a differentiation marker SSEA1 could be detected after four days of cultivation in the suspension bioreactor. The result is based on the analysis of 10,000 cells. n = 3 biological replicates. The error bars represent the standard deviation (±SD).
3D microcarrier cell culture in CERO bioreactors
In the experiments, hiPSC lines UKBi005-A was used (https://hpscreg.eu/cell-line/UKBi005-A). According to a standardized protocol, cells were cultivated in Matrigel™-coated petri dishes in mTeSR™1 medium (Stemcell Technologies, France) and split at 60-80% confluency using enzyme-free detachments reagent EDTA. The 3D cultivation was carried out on alginate microcarriers coated with Matrigel™ in the suspension bioreactor CERO. The alginate microcarriers modified with Matrigel™ were first prepared for the inoculation of the cells. Microcarrier with a total growth area of 20 cm2 were used for cultivation in the suspension bioreactor. The alginate microcarriers were taken up in 1 ml medium consisting of mTeSR1 and 10 mM ROCK inhibitor (at a ratio of 1:1000). A seeding density of 6.0 - 104 cells/cm2 cell scaffold area was used for cultivation in the suspension bioreactor. After cell counting, hiPSC were mixed with the microcarrier in cell culture medium and transferred into CERO-tubes. The tubes were subsequently placed into the CERO bioreactor system (both OMNI Life Science GmbH & Co. KG, Germany) and the inoculation program was carried out for 12h (rotation speed: 40 rpm, rotation period: 4 s, agitation period: 2min, agitation pause: 5 min). After 12h, the bioreactors were rotated during cultivation sequence with 40 rpm and a rotation period of 4 s.
Cell harvest and cell analysis
For cell harvest, the microcarriers were washed twice with phosphate buffered saline, whereby the buffer was added and aspirated again after a short incubation. After the second washing step, EDTA solution was applied. The tube with the microcarriers was placed in the suspension bioreactor and incubated for 20 min using an appropriate programme. The tubes were rotated at 60 rpm with a constant change of direction (every 5 s) and without pause. At the end of the incubation time, the tube was removed from the suspension bioreactor and shaken once. To stop the detachment reaction, a medium consisting of mTeSR1 and 10 mM Rock inhibitor (in a ratio of 1:1000) was pipetted into the cell suspension. To separate the alginate microcarriers from the iPS cells, the cell suspension was filtered through a 200 μm sieve. After filtration, the total cell number and the viability of the cells were determined. QC samples were then taken for FACS analysis. The cell count and viability of the iPS culture was determined using the NucleoCounter® NC-202™ automated cell counter. Two measurement methods were used to analyse the expression of pluripotency and differentiation markers. Throughout the study, the pluripotency of the UKBi005-A cell line was assessed using flow cytometry and phase contrast microscopy.
Results and Discussion
General properties and handling of alginate microcarriers
The growth area of the alginate microcarriers can be adjusted by pipetting and centrifuging the corresponding volume. Each vial indicates the growth area per millilitre, enabling a quick and dependable inoculation process. The microcarriers are ready-to-use and stored in isotonic NaCl solution at 4°C until use, eliminating the need for rehydration. The microcarriers are compatible with poloxamers at low concentrations. This minimises adsorption of the microcarriers to plastic surfaces. HiPSC on transparent, non-auto-fluorescent alginate microcarriers can be imaged for documentation purposes and analysed using various microscopic techniques, such as phase contrast or confocal laser scanning microscopy. Fluorescent dyes and antibodies can be used for staining, and imaging can be done without modifying the manufacturer's staining protocol. Alginate microcarriers, unlike other commercial microcarriers, are soft and can imitate the mechanical properties of the in vivo environment. The diameter of the microcarriers is adjustable, and microcarriers ranging from 100 µm to 500 µm can be produced. Standard laboratory equipment can be used for cell harvest when working with these microcarriers. Separating the medium and microcarriers overgrown with cells can be achieved through centrifugation and sieving. Alginate microcarriers have a density of about 1014 kg/m³, which causes them to sediment slowly. However, this also means that less energy is required for stirring in stirred bioreactors. Cell detachment can be achieved using trypsin, TrypLE™, accutase, or EDTA. Additionally, commercially available alginate lyases can be used to degrade hydrogels, allowing for the recultivation of expanded cells on standard 2D cell culture surfaces. The viscosity and elastic modulus of alginate change over time, depending on storage conditions and production mould. Varying the geometry and modulus (2.0-8.0 kPa) of the cell scaffold does not affect the efficiency or quality of hiPSC-3D cultivation.
3D expansion of hiPSC in CERO bioreactors
The alginate microcarriers were used to expand hiPSC in CERO suspension bioreactors. Microcarriers with different mechanical properties (increasing E-Modul) have been studied and after 4 days viability and total cell number has been measured. Overall, the data (see Figure 2 a-c) show an excellent compatibility with hiPSC independent from the alginate’s formulation. The viability after expansion was greater than 85% and a total cell number of at least 6.0x106 ± 1,8x106 cells was achieved. The results lead to the conclusion, that there are no adverse effects of different moduli of elasticity on the proliferation of UKBi005-A cells. Neither the total cell number nor the viability were affected by the mechanical properties of the cell scaffold. The expanded cells were also analysed by flow cytometry (FACS). No deviations in the expression pattern of the pluripotency markers could be detected as a function of the Young's modulus of the cell scaffolds. In addition to the qualitative evaluation of the proliferation and pluripotency of the UKBi005-A cells, a microscopic evaluation was also carried out during the entire cultivation process. The representative images from last day of cultivation are shown in Figures 3.
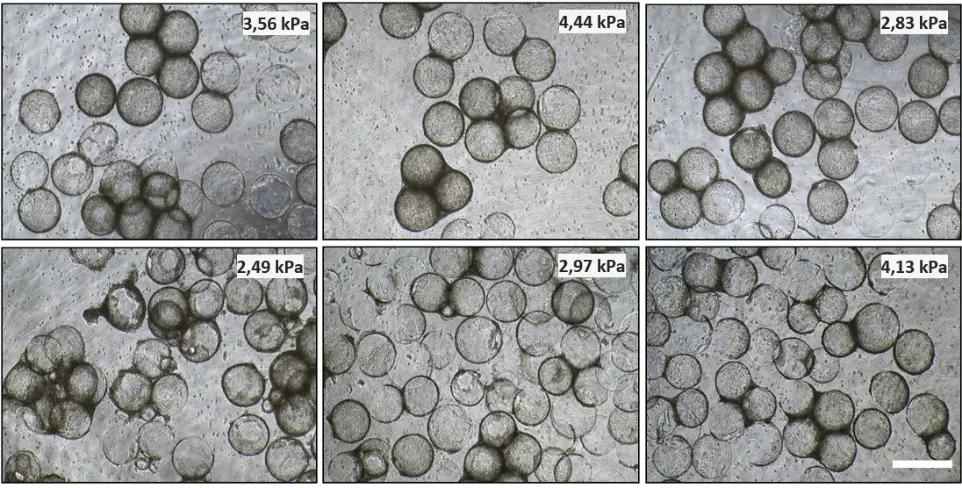
Figure 3: Cell adhesion and proliferation. Representative microscopic images after the fourth 3D cultivation day (96 h, immediately before harvest) of UKBi005-A cell line in the suspension bioreactor on alginate cell scaffolds prepared from differently composed alginate mixtures. The white scale bar corresponds to 500 µm for all images.
Conclusions
In this study, alginate microcarriers coated with Matrigel™ were characterised as an important biomaterial for stem cell expansion in suspension bioreactors on soft microcarriers. New insights were gained into the mechanical properties of alginate under the influence of environmental conditions, the correlation between viscosity and elastic modulus, and the interaction between alginate and iPSC lines. It was found that despite the different viscosities of the mixture components, the alginate microcarriers had a modulus of elasticity of 2.5 to 4.5 kPa, which did not affect the efficiency of hiPSC expansion. The hiPSCs cultured on these cell scaffolds showed high viability (85-95%) and excellent adhesion. In additional studies could be demonstrated that the alginate microcarriers can be part of complex stem cell processes (see [7]) and are compatible with various human cell systems. Thus, the alginate microcarriers can be integrated into cultivation protocols of mesenchymal stem cells [1] and expansion and differentiation protocols of hiPSC for renal proximal tubular epithelial cells [5], neural cells [3], and 3,56 kPa 2,83 kPa 2,49 kPa 4,44 kPa 2,97 kPa 4,13 kPa
the cultivation of cardiomyocytes. Furthermore, the alginate microcarrier has emerged as stable in vitrification studies of adherent hiPSC [6]. The key benefits of UHV-alginate microcarriers in stem cell processes are (not an exhaustive list):
- Adjustable soft, physiological surfaces
- Adjustable diameter
- Various coatings (Matrigel™, collagen I, laminin, tyramine, etc.)
- Adjustable mechanical properties (brain-like to heart-like tissue)
- Multidimensional biological interactions
- Compatibility to various cell systems (hMSC, hiPSC, cardiomyocytes, neural cell, fibroblasts, endothelial cells, …)
- Easy scalability
- High surface to volume ratio
- Easy integration in existing processes
- Easy recovery of overgrown microcarriers e.g. by sieving
- Enzymatic degradation using alginate lyases
- Transparency allows microscopic analysis
Alginate Microcarriers for Stem Cells – Request a Free Demo Today
Download Alginate Microcarriers Application Note
Scale up your Alginate microcarriers research with CERO 3D. Achieve efficient results with a streamlined, cost-effective workflow. The CERO 3D Incubator & Bioreactor enables automated, microcarrier-free 3D culture, ensuring homogeneous cell aggregates and robust scalability.
Optimize your Alginate microcarriers workflows with CERO 3D. Request a free demo today!
Request a Free Demo
References
[1] Gepp, M.M., Fischer, B., Schulz, A., Dobringer, J., Gentile, L., Vásquez, J.A., Neubauer, J.C., and Zimmermann, H. 2017. Bioactive surfaces from seaweed-derived alginates for the cultivation of human stem cells. J. Appl. Phycol. 29, 5, 2451–2461.
[2] Schulz, A., Gepp, M.M., Stracke, F., Briesen, H. von, Neubauer, J.C., and Zimmermann, H. 2019. Tyramine-conjugated alginate hydrogels as a platform for bioactive scaffolds. J. Biomed. Mater. Res. A 107, 1, 114–121.
[3] Kwok, C.K., Sébastien, I., Hariharan, K., Meiser, I., Wihan, J., Altmaier, S., Karnatz, I., Bauer, D., Fischer, B., Feile, A., Cabrera-Socorro, A., Rasmussen, M., Holst, B., Neubauer, J.C., Clausen, C., Verfaillie, C., Ebneth, A., Hansson, M., Steeg, R., and Zimmermann, H. 2022. Scalable expansion of iPSC and their derivatives across multiple lineages. Reprod. Toxicol. 112, 23–35.
[4] Zimmermann, H., Zimmermann, D., Reuss, R., Feilen, P.J., Manz, B., Katsen, A., Weber, M., Ihmig, F.R., Ehrhart, F., Geßner, P., Behringer, M., Steinbach, A., Wegner, L.H., Sukhorukov, V.L., Vásquez, J.A., Schneider, S., Weber, M.M., Volke, F., Wolf, R., and Zimmermann, U. 2005. Towards a medically approved technology for alginate-based microcapsules allowing long-term immunoisolated transplantation. J. Mater. Sci.: Mater. Med. 16, 6, 491–501.
[5] Ngo, T.T.T., Rossbach, B., Sébastien, I., Neubauer, J.C., Kurtz, A., and Hariharan, K. 2022. Functional differentiation and scalable production of renal proximal tubular epithelial cells from human pluripotent stem cells in a dynamic culture system. Cell Prolif., e13190.
[6] Meiser, I., Majer, J., Katsen-Globa, A., Schulz, A., Schmidt, K., Stracke, F., Koutsouraki, E., Witt, G., Keminer, O., Pless, O., Gardner, J., Claussen, C., Gribbon, P., Neubauer, J.C., and Zimmermann, H. 2021. Droplet-based vitrification of adherent human induced pluripotent stem cells on alginate microcarrier influenced by adhesion time and matrix elasticity. Cryobiology 103, 57–69.
[7] Neubauer, J.C., and Gepp, M.M. 2022. Biomaterialien — Nachbildung der Stammzellnische in Bioreaktoren. Biospektrum 28, 5, 501–503.